Turning up the heat: How thermally conductive silicones aid solar and storage development
Solar inverters and battery energy storage systems (BESS) are key components in the global transition to clean energy. As demand for solar and storage grows, the companies that design and manufacture inverters and batteries need safe, cost-effective and energy-efficient materials that support high-volume production. Thermally conductive silicones are meeting these and other challenges, which include resistance to high heat, the risk of fire and exposure to dust, moisture and humidity. Solar designers have a choice of materials, but advanced silicones provide important advantages.
Material considerations and comparisons
When electronics overheat, their performance suffers. There’s also the risk of fire, a leading safety concern. Both inverters and BESS generate significant amounts of heat, which means they need materials that can withstand extreme temperatures and guide heat away from sensitive electronics. Thermally conductive silicones resist high temperatures, dissipate heat and provide flame resistance. They also resist dust, moisture and humidity and tolerate low outdoor temperatures.
Thermally conductive silicones are available in a variety of forms, including adhesives, encapsulants, gap fillers, gels, conformal coatings, greases and sealants. The addition of specialized fillers to the base elastomer enables these advanced materials to move heat away from important electronics while retaining the inherent advantages of silicones.
By filling the gaps between a heat source and a heat sink, thermally conductive silicones prevent the ingress of moisture and replace the air that would otherwise fill these voids. This is important for thermal management because air has a significantly lower thermal conductivity (TC), a measure of a material’s ability to conduct heat. Thermally conductive silicones also have a higher TC than organic materials such as polyurethane (PU), which is traditionally used.
Even when they contain fillers, silicones are inherently flexible and stress-relieving at elevated temperatures. That is important because the electronic materials used in inverters and BESS, such as plastics and metals, expand and contract at different rates. Although silicones are not as strong as epoxies, they are not as prone to cracking when materials have different coefficients of thermal expansion (CTE).
For manufacturers, thermally conductive silicones support automated mixing and dispensing for high-volume production. In addition, these advanced materials cure, or achieve their final properties, at room temperature instead of with added heat. This provides an energy savings, but inverter and BESS manufacturers can also use ovens for accelerated curing.
Thermally conductive silicones for BESS
Lithium-ion batteries are commonly used for battery energy storage systems because they are cost-effective. However, lithium batteries are susceptible to thermal runaway, which causes the cells in a battery to overheat uncontrollably. To reduce the risk of fire, thermally conductive silicones are used with either cylindrical or prismatic cells.
In batteries with cylindrical cells, thermally conductive adhesives are used to attach individual cells to a substrate. Thermally conductive silicone encapsulants are also used, but to enclose or encase cells instead. These fire-resistant encapsulants provide electrical isolation, stress relief and vibration damping. Because of their low viscosity, they flow readily and can fill voids.
Thermally conductive silicones also provide adhesion and component staking in battery packs with prismatic or pouch cells. Staking protects and supports cells that could be damaged by shock or vibration. Instead of encapsulants, thermally conductive gap fillers can be used to manage heat and fill voids. These soft, compressible materials spread easily and remain in place during product assembly.
Although thermal silicone gap fillers have limited adhesion, they offer key benefits. For example, they combine stress relief with vibration damping. They are suitable for gap tolerances ranging from 150 µm to 5 mm and provide excellent interfacial contact for dependable cross-joint strength. These materials also support fast, accurate dispensing without afterflow and cure at room temperature. Plus, they can be removed for battery pack repair or recycling.
In addition to thermally conductive silicones, foam technology is used battery cells. With their high-temperature resistance, advanced silicone foams provide protection against thermal runaway, a chemical reaction that occurs when the temperature inside a battery increases rapidly and poses the risk of fire. Silicone foams also support vehicle lightweighting with EV manufacturing.
Thermally conductive silicones for PV inverters
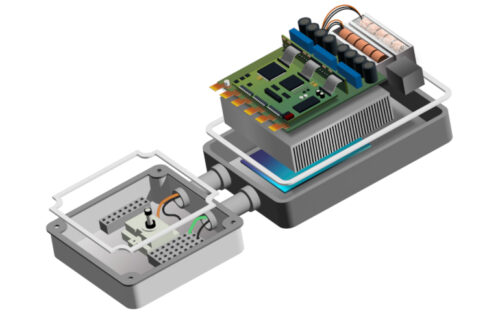
PV inverter illustration, showing all the internal components to showcase where Dow products are used.
For all types of inverters used in solar systems, thermally conductive silicone encapsulants, greases, gels and other products are used. The service life of an inverter is usually 10 to 15 years, but material selection supports long-term performance. Organic materials such as polyurethanes are subject to higher stresses under thermal cycling, which can lead to cracking and premature device failure. Research from the National Renewable Energy Laboratory (NREL) demonstrates that PV inverter modules that use silicone encapsulants have lower degradation rates than inverters that use encapsulants with other chemistries.
In inverters, thermally conductive silicone encapsulants fill the gaps between electronic components and the enclosure for the inverter’s inductance module. Sometimes, these modules feature complex, hard-to-fill geometries. With the aid of static mixers, these advanced materials are easy to dispense and can fill complex voids.
Thermal silicone greases are also used with inverters, typically to achieve thinner bond lines. These greases are easy to rework and, depending on their formulation, have viscosities ranging from semi-flowable to non-flowable. Application methods include needle dispensing, squeegees and screen printing.
Thermal silicones gels are used to protect the IGBTs in inverters. These advanced materials offer limited adhesion but have thermal conductivities ranging from 1.5 to ~6 W/mK. Among their advantages, thermal silicone gels are stress-relieving. Because they are flowable, they can be applied with high precision for greater yields.
Other thermally conductive silicones for PV inverters include conformal coatings, sealants and staking adhesives. Typically, conformal coatings are used with high-current printed circuit boards (PCBs), gate driver boards, user interface boards and communications PCBs. Silicone sealants are applied to inverter enclosures to help exclude dust and water. Thermally conductive staking adhesives can replace mechanical fasteners and bond well to a wide range of materials.
Promoting more efficient and sustainable manufacturing processes
As the world transitions to renewable energy, inverters and BESS are becoming essential technologies. Thermally conductive silicones can meet challenging design requirements, but they also support fast, energy-efficient and cost-effective manufacturing. The fact that so many of these materials are reworkable also helps to reduce waste. For designers and manufacturers alike, choosing thermally conductive solutions can support a more sustainable future.
Cody A. Schoener, PhD, is Marketing Manager for Dow Performance Silicones at The Dow Chemical Company. In this role, he is serving the Consumer Electronic, Industrial Electronic, and Pressure Sensitive Industry. His responsibilities include short and long-term strategy development, innovation portfolio management, and leading a global team specifically for industrial electronic strategy and global accounts. Prior to his role in Marketing, he spent 8 years as a technical and developmental scientist for The Dow Chemical Company servicing polyurethane and cellulosic chemistries. He received his BS and MS degrees in Biomedical Engineering from Texas A&M University, College Station, Texas, in 2007 and 2009, respectively. He earned his PhD in chemical engineering from University of Texas at Austin in 2012.